In a lab at the University of Waterloo’s Institute for Quantum Computing (IQC), two contraptions consisting of a number of metal containers, poles and beams surrounded by thick, orange wires emit a nonstop, metallic jangling sound. Called dilution refrigerators, these cryogenic devices are using helium isotopes to cool down a handful of tiny – about one centimetre by one centimetre – aluminum circuits to one one-hundredth of a degree Kelvin. At this rock-bottom temperature, the aluminum loses its electrical resistance, the atoms slow down and quantum mechanics takes over.
Quantum mechanics has been described as “spooky” by Albert Einstein. Bill Gates calls quantum calculations “hieroglyphics.” And one of the pioneers in the field, the late American theoretical physicist Richard Feynman, once remarked, “I think I can safely say that nobody understands quantum mechanics.” Yet it’s the quirky and confusing behaviours in the quantum realm that Christopher Wilson, a member of the IQC and a professor in the department of electrical and computer engineering, and his team at U of Waterloo are eager to observe and harness.
The IQC has over 200 researchers from a range of disciplines working on quantum projects, many of them with links to computing. Nationwide, a few hundred more Canadian academics are exploring other facets of quantum information, both experimental and theoretical. They’re collaborating with companies such as IBM and Google, plus the start-up sector, but are also vying to be contenders in the worldwide race to build a large-scale, useful quantum computer. Such a computer, they say, would dramatically outperform traditional ones in a range of applications.
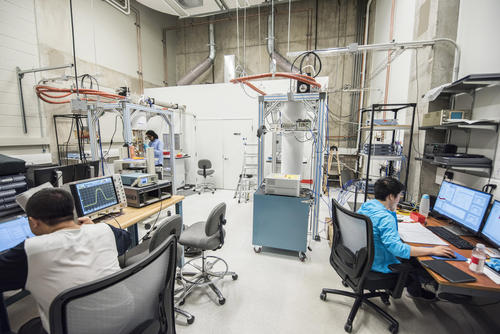
“If we can make it work, and that’s a big if, the computational power will be revolutionary,” says University of Toronto physics professor Daniel James. “If you look at social history over the last hundred years and how computers have changed lives, this is going to be equally as disruptive a change.”
A quantum computer can process information in new ways, solving previously impossible computational problems. This is because of quantum mechanics’ unique qualities, including superposition, which means that a quantum system can be in multiple states at the same time. While a computer bit is either a 1 or a 0, a quantum bit – or qubit – can be partially 1 and partially 0 simultaneously. Layer that with entanglement, where two or more particles can link up in unison, even if they are far from each other.
But quantum phenomena only happen in tiny particles such as atoms, electrons and photons. Once components get more complicated, the rules of classical physics take over. (“But where does this transition from quantum to classical happen?” Dr. Wilson asks rhetorically. “This is unknown.”) What’s more, if you observe a quantum system or try to interact with it, it will decohere – i.e., return to a classical state – and stop its unique behaviours. Vibrations or magnetic fields or any so-called “noise” can trigger decoherence, leading to errors. Plus, since you can’t observe quantum calculations partway through, it’s difficult to debug and check for errors. In other words, quantum computing is hard, and we’re not there yet.
One thing seems sure: the race promises a serious windfall at the finish. That may go to a large American or Chinese company with the resources to build ambitious computers, but Canadian academics are banking on there being multiple victors. “There are actually several races,” says Michele Mosca, co-founder of the IQC and a professor of mathematics at U of Waterloo. Quantum information science, as a larger field, has many uses, such as in sensors already employed today in healthcare scanners. Quantum communications, including a quantum internet, could be here soon, too, plus there’s cryptography and simulations. “There are so many spin-off technologies. Your work is never wasted,” says Alexandre Blais, scientific director of the Institut quantique at Université de Sherbrooke. Here’s how Canadian academics plan to come out winners, or at least top-ten finishers, in the quantum race.
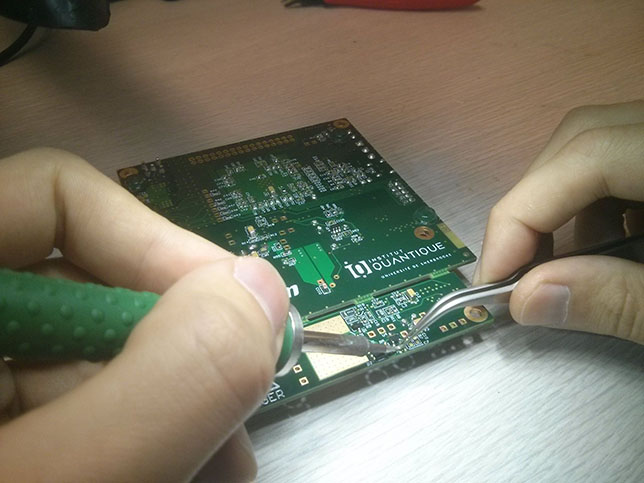
Once, there was only classical physics to explain the Big Bang, relativity and the like. Then, in 1900, German theoretical physicist Max Planck coined the term quanta to describe the tiny packets of light that glow at different colours at various temperatures. Over the next few decades, physicists, including famous thinkers such as Einstein and Erwin Schrödinger, cobbled together the theories behind quantum mechanics, or how physics happens differently at the microscopic level.
Starting in the 1960s, the basics of quantum computing emerged, with a model proposed for one in 1980. In 1984, Gilles Brassard at Université de Montréal co-invented BB84, a protocol for leveraging quantum mechanics for cryptography. Dr. Brassard is widely considered a pioneer of quantum information science. He’s still at U de Montréal, where he holds the Canada Research Chair in Quantum Information Science and continues to publish widely. A decade later, mathematician Peter Shor came up with an algorithm that showed that a computer built on quantum mechanics could break the type of cryptography used to secure data transmissions. “This is what got people thinking about quantum computing,” says Dr. Wilson.
Dr. Mosca recalls that he, Dr. Brassard and a small handful of Canadian academics landed an $86,500 collaborative grant for a quantum project from the Natural Sciences and Engineering Research Council in 2000. “I have no idea what the grant panel thought,” Dr. Mosca says now, “but there was no proactive effort in quantum computing at the time. It was not a ‘priority area’ anyway.” Nevertheless, hopes were high. In 1999, one of the first Canadian quantum start-ups, D-Wave, was launched by a trio out of the University of British Columbia.
Then, in 2002, Dr. Mosca and fellow physicist Raymond Laflamme founded the IQC with funding from Blackberry founder Mike Lazaridis. A teenaged Stephanie Simmons read about the new institute in the local paper. “I thought it was the coolest thing,” she recalls, and based her plan to attend U of Waterloo on that article and the timeline it implied. “I was under the impression that quantum computers would be commercially available by the time I was done my undergrad,” says Dr. Simmons, now an assistant professor of physics at Simon Fraser University, working on quantum technology.
As the field grew, Canada came out as an early leader, with federal agencies investing about $1 billion over the past decade. In 2010, UBC founded the Quantum Matter Institute, which got a $66.5-million boost five years later from the Canada First Research Excellence Fund; U de Sherbrooke’s Institut quantique received $33.5 million from the fund. Smaller research centres also popped up across the country. In 2019, SFU landed $17 million in provincial funding to start the Quantum Algorithms Institute.
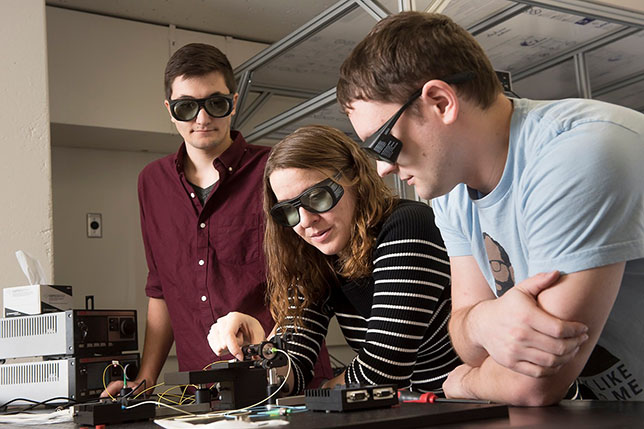
Meanwhile, academics began to connect with the commercial sector. The University of Toronto launched a quantum stream for its Creative Destruction Lab accelerator in 2017, while IBM has partnered with and funded projects at U of Waterloo. Toronto-based quantum start-up Xanadu, which has landed $45 million in venture capital and runs an eight-qubit computer, works with professors from U of T.
In October 2019, the race sped up when Google and the University of California, Santa Barbara, claimed quantum supremacy by revealing that their 53-qubit computer Sycamore had performed a calculation in 200 seconds that would take a classical supercomputer 10,000 years to accomplish. Rival IBM pooh-poohed the claim, saying the (not very useful) calculation could have been done in just 2½ days. “Whether we accept Google’s argument or not is not important,” says Barry Sanders, a professor and director of the Institute for Quantum Science and Technology at the University of Calgary. “What’s important is we’re around the turning point where quantum computers will soon be able to do things that are better than classical computers.”
“What’s important is we’re around the turning point where quantum computers will soon be able to do things that are better than classical computers.”
Despite Sycamore’s antics, reliable, error-controlled, large-scale quantum computers remain elusive. California Institute of Technology professor John Preskill has dubbed this the noisy intermediate-scale quantum era. Error correction entails using computer algorithms to detect and fix computational mistakes, but you need many qubits to make strong error-correcting codes. While universities work with companies who build increasingly large devices, on campus they often concern themselves with perfecting the basic science for the next generation of devices.
“The big problem on the theory side stands: what can we do with these quantum computers? How do we use the quantum for computation?” asks Robert Raussendorf, an associate professor in the department of physics and astronomy at UBC. David Gosset, an IQC member and an associate professor in the department of combinatorics and optimization at U of Waterloo, says theorists struggle to create useful algorithms. “It’s hard to predict where things are going to go and what new ideas are needed,” he says.
While quantum computers exist, the best approach for building them and how to make them function have yet to be firmed up. B.C.-based D-Wave uses a process called quantum annealing to run its quantum computers. The company boasts that its newest device, accessible via the cloud, will run with 5,000 qubits. D-Wave uses an open-source platform and its customers have built over 200 early-stage applications for a variety of uses. The company is even offering to apply its quantum computing resources to researchers’ COVID-19 projects.
Most quantum companies leverage superposition and entanglement, and the hardware of choice for Google, IBM and other big players is superconducting chips (D-Wave does use them as well). But these aren’t perfected yet, and they’re expensive. Dr. Wilson at IQC has roughly four or five qubits working together, and for one line of research his team is seeing if they can use quantum entanglement to make better radar or general sensors.
At SFU, Dr. Simmons thinks silicon chips are the way to go; silicon is already being used to make computer chips for classical computers, so it’s readily available. “The old, solid-state options have quantum lifetimes that are microseconds, [but] we are getting lifetimes lasting hours,” she says. Experiments at SFU include linking silicon with qubits made of photons via lasers. “This approach could underpin the entire eventual quantum internet,” says Dr. Simmons. You can see photonics at work at Toronto company Xanadu, and at numerous university labs as well. Computing happens at room temperature, which is a benefit over chilled superconducting chips.
Another branch of photonics involves the use of trapped ions, which are charged atomic particles held in an electromagnetic field and manipulated with a laser. Dr. James, who works on the theoretic side of this work with a team at U of T, says you can scale up to more qubits and control the particles, but they’re still fussy to work with – as are most quantum-friendly materials. “You want a computer that you can turn on in the morning and have it work. But that’s difficult to do with these kinds of technologies,” says Dr. James.
Meanwhile, some academics are looking past the building blocks of this new era of computers and studying their disruptive repercussions. “Let’s be ready when quantum computers come,” says Dr. Mosca. “We want our banks and the like to be ready.” His research focuses on how to make sure quantum computers’ ability to crack cryptography doesn’t lead to data hacks and shutting down critical systems, such as hospitals. He advocates for governments and industry to make themselves quantum-ready.
No one knows when we’ll have a pristine, fully functioning quantum computer. But, whenever it is, it’s likely that few people will own one. “It’s not as though your laptop is going to be replaced by a quantum computer,” says Dr. James. Expect these expensive and likely delicate contraptions to be owned by big companies – and maybe a few universities – while clients pay to connect to them via the cloud. By then, other quantum information technologies will impact a wide range of industries. Sensing will be better and smarter, and perhaps we’ll have a quantum internet. A blend of quantum computing and quantum simulations could, in time, allow the pharmaceutical industry to zero in on drug targets. Indeed, many processes that happen at the quantum level, like human biology, may be best simulated using quantum technologies. As these evolve, expect widespread disruption. “We’re talking about a deep change in society,” says Dr. Blais of U de Sherbrooke.
While the wealthy, commercial players may win most of the high-profile heats in the quantum race, Canadian academics hope to still make an impact through patents and spin-off companies. “We can’t just become complacent. We can’t keep giving away our intellectual property,” says Dr. Mosca. He patents any work he thinks has potential future value – it’s hard to guess which ideas will be useful, he admits – and worries that his colleagues across the country don’t hold onto their IP rights. He thinks software and its applications hold the most promise for monetization for Canadian start-ups, and wants to see more support for these efforts.
Indeed, keeping Canada a contender will depend on sufficient funding and a stronger support network. To that end, a group of academics and industry representatives have been developing a national quantum strategy and are hoping to secure federal funding. Universities have an important role to play in terms of the basic science, but they will also be needed to train the next generation of people to make, fix and operate these wonderful new devices. “Industry already needs more people than we have,” says Dr. Gosset.
Indeed, not just physics but also engineering, math and computer science are thriving because of the promise of a quantum future. They’ve got research questions to last years, and much to teach students who will be able to find jobs in a range of sectors. And while it’s very difficult to fully fathom the quantum realm, it’s an exceptionally promising field for the next generation of scientists. “Everyone’s welcome to the party,” says Dr. Sanders of U of C. “There’s enough room for everyone to do their thing.”